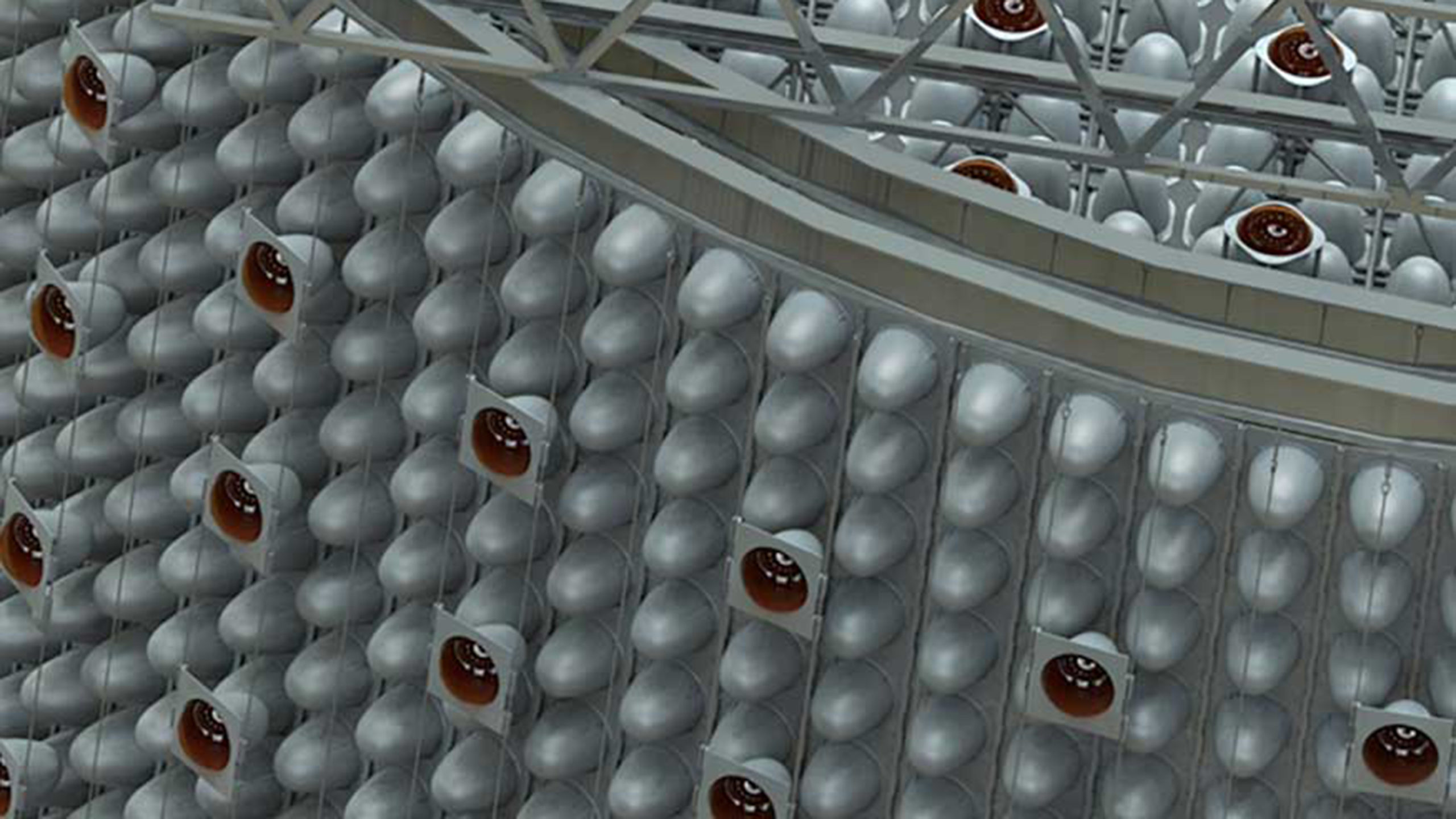
Can tiny, invisible particles help stop the spread of nuclear weapons?
The key to preventing nuclear proliferation may depend on a little bit of ghost hunting.
Scientists have long been interested in a device that can detect neutrinos, ghost-like particles that have no electric charge and nearly no mass — and therefore can pass through matter. Now, researchers are closer than ever to deploying technology that can spot those elusive subatomic particles and, in doing so, alert international authorities to the illicit production of plutonium, a key fuel for nuclear bombs.
The technology may provide a “way to monitor the plutonium content in a nuclear reactor in real time that we just don’t have right now,” said Bethany Goldblum (M.S.’05, Ph.D.’07 NE), a top researcher with UC Berkeley’s Department of Nuclear Engineering.
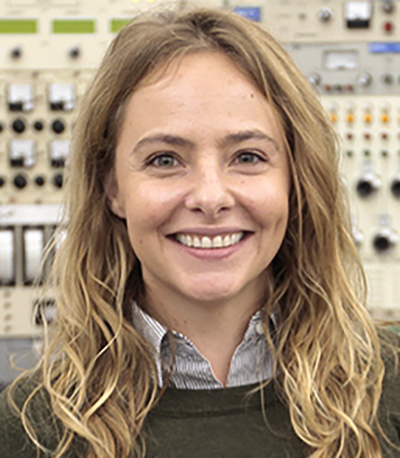
Bethany Goldblum, a researcher in the Department of Nuclear Engineering, is also the executive director of the Berkeley-based Nuclear Science and Security Consortium
Goldblum, the executive director of the Berkeley-based Nuclear Science and Security Consortium, co-wrote a study published this week in the Review of Modern Physics that examines the feasibility of neutrino detectors in nuclear nonproliferation efforts. The study’s co-authors include Adam Bernstein and Nathaniel Bowden from Lawrence Livermore National Laboratory, Patrick Huber from Virginia Tech, Igor Jovanovic from the University of Michigan and John Mattingly from North Carolina State University.
The study ultimately concludes that such technology deployed outside nuclear reactors could prove effective in ensuring that countries are not making weapons-related material under the guise of peaceful civilian energy production. The report also advances the idea that researchers could one day use the technology to discover or exclude the presence of reactors at distances of a few hundred kilometers.
“Over several decades, physicists have conceived many ideas for using fission neutrinos in nuclear security,” the study says. “Some ideas remain in the realm of pen and paper, constrained by basic physical and practical considerations. For other concepts, demonstrated technology is catching up with real opportunities.”
The ghost particle
Neutrinos are the most abundant particles in the universe, having been formed by large nuclear explosions like the Big Bang, supernovas and the fusion process that happens inside the sun. They travel near the speed of light, have little mass and carry no electric charge.
Because of these attributes, neutrinos can pass through matter and are incredibly difficult to detect, which is why scientists often refer to them as “ghost particles.” For example, if 10 trillion neutrinos struck the Earth, all but one would pass through the planet without having interacted with anything at all.
In 1956, Clyde Cowen and Frederick Reins, two scientists at the Los Alamos National Laboratory in New Mexico, confirmed the neutrino’s existence, work that eventually earned the Nobel Prize in Physics. The duo placed two large water tanks near a nuclear reactor, which produces electron antineutrinos in huge quantities, as part of the fission process.
As it turns out, neutrinos can collide with protons in the water and produce a neutron and a positron through a process called inverse beta decay. When the positron moves through the water, it produces a flash of light that special sensors can detect.
Up to this point, scientists were primarily interested in finding neutrinos because the particles might offer clues to the universe’s origin and the formation of stars and galaxies. But starting around the turn of the 21st century, the idea that neutrino detectors could be used in nuclear nonproliferation efforts started to gain real traction.
In 2000, Adam Bernstein, then a postdoctoral fellow at the Sandia National Laboratory in Livermore, California, wrote a paper exploring the idea of using detectors filled with purified water to spot neutrinos produced from nuclear explosions.
In many ways, water is a great medium to detect neutrinos because it is easy to purify, cheap and is transparent to light produced by neutrinos colliding with water molecules. The key would be to build detectors big enough to hold enough water to see the neutrino signal above background radiation.
However, finding neutrinos in water is still pretty hard. Bernstein found that adding small amounts of gadolinium — a rare earth metal with unusual nuclear properties — to the water could significantly boost the detector’s chances of spotting neutrinos. In gadolinium-doped water, neutrino interactions produce a much stronger signal than neutrinos in water alone.
Bernstein eventually abandoned the idea to monitor explosions because the cost and size of such neutrino detectors would make the technology impractical, especially compared to existing, cheaper technologies like seismic detectors, he said.
Instead, Bernstein turned his attention to using the gadolinium-doped technology to catch neutrinos from nuclear reactors.
“Since we’re still mostly using water, it is possible to build large detectors, up to 100 kilotons in size or more, to spot these reactor neutrinos,” said Bernstein, now a staff physicist at the Lawrence Livermore National Laboratory (LLNL) and director of the lab’s Rare Event Detection group in the Nuclear and Chemical Sciences division.
“The neutrino signature would stand out much more readily above background radiation even in a big detector,” he said.
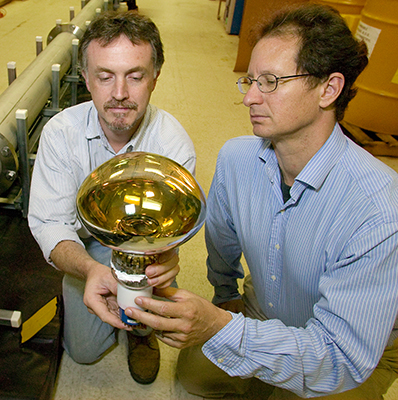
Adam Bernstein (r) and Steve Dazeley examining one of the 8″ diameter photomultiplier tubes that are used to collect light generated by antineutrino interactions in both the water and scintillator detectors. (Photo courtesy of the Lawrence Livermore National Laboratory)
LLNL is the lead laboratory for a proposed United States/United Kingdom experiment, called WATCHMAN, to demonstrate remote monitoring of nuclear reactors using a kiloton-scale antineutrino detector.
This experiment has already “exceeded my expectations,” Bernstein said. “The idea that the nonproliferation community might one day be able to use this technology that until now has been the exclusive province of fundamental science is an exciting motivation for this work.”
Halting the spread of nukes
Since 1970, nearly 200 nations signed the landmark Treaty of the Non-Proliferation of Nuclear Weapons (NPT), which seeks to limit the spread of nuclear weapons.
Through a combination of remote monitoring and on–the–ground inspections, containment and surveillance, the International Atomic Energy Agency (IAEA) commands plenty of tools to figure out if countries are using nuclear energy for peaceful purposes, Goldblum said.
But what happens if the line between civilian and military use of nuclear energy is not so clear? For example, the United States has long accused Iran of trying to make nuclear weapons, but Iran says it wants to develop nuclear capabilities for civilian power generation.
The knowledge to construct a nuclear bomb is actually pretty well known. The hard part is getting enough materials — either enriched uranium or plutonium — to fuel the weapon. A country can reprocess the spent fuel from a civilian nuclear reactor and extract plutonium for a weapon. And a nuclear bomb only requires about 10 kilograms of plutonium.
The so-called “dual-use” capabilities of nuclear reactors presents a significant challenge to the IAEA.
“None of the countries now embarking on civil nuclear power programs say they are planning to acquire reprocessing capabilities,” according to a 2017 report by the Brookings Institute think tank.
“But many of them are unwilling to forswear what they consider to be their ‘right’ eventually to have dual-use capabilities.”
The neutrino detection technology could offer a solution. In addition to the large systems like WATCHMAN, scientists have constructed much smaller detectors that can be deployed close to reactor cores — provided operators allow such access.
Optimizing reactor power levels to produce plutonium, a telltale sign that a country is trying to build a bomb, will change the rate and energy spectrum of antineutrinos that a device parked outside of the reactor can detect.
And since these particles can pass through matter, the operator can’t shield the reactor’s release of antineutrinos the same way lead blocks X-rays. So if a country wants to operate a civilian nuclear power program, an antineutrino detector could provide an effective tool to continuously verify the reactor is only producing energy for peaceful purposes.
For now, a detector must stay within tens of meters of the reactor to be effective. But in the future, could such technology spot antineutrinos from longer distances and even across borders?
For distances 100 kilometers or beyond, the Review of Modern Physics study shows detectors would need to be 10 to 100 times bigger than WATCHMAN. But researchers hope WATCHMAN will demonstrate the basic technology and provide a platform for study of a range of possible enhancements to improve standoff and overall sensitivity.
And in any case, the mere knowledge that such technology has become a reality could prove to be a powerful deterrent to nuclear proliferation in itself.