Cellular Connections
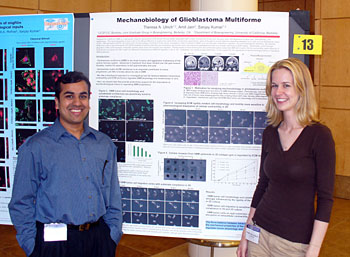
Sanjay Kumar’s lab is studying glioblastoma multiforme in a promising new way.
No cancer is good, but brain cancers are among the worst. The most common type, glioblastoma multiforme (GBM), can strike at any age and is usually fatal. What makes GBM so deadly is how rapidly it spreads, sending tumor cells deep into the surrounding tissue. As a result, it almost always grows back after any treatment, including surgery.
“This form of cancer has a very bad prognosis,” explains bioengineering professor Sanjay Kumar, “Even with aggressive treatment, its average survival time from diagnosis is around one year.”
Kumar’s lab is studying GBM in a promising new way. Instead of using the traditional biochem approach of culturing tumor cells in liquid and tracking their chemistry, Kumar’s lab is reverse-engineering the cells’ mechanics, deciphering how they interact with their physical environment. Since cell mechanics underlie the tumor’s spread, this approach is key to understanding how this heartbreaking disease progresses and to developing new forms of therapy that might halt it.
“Individual cells detach and migrate away from the main tumor to establish themselves in surrounding brain tissue, making the tumor almost impossible to completely remove surgically. Very little is known about how the physical environment controls GBM cell invasion and growth, and we think this is an important and interesting problem.”
Kumar’s lab focuses on the cell’s cytoskeleton, the system of molecular-scale scaffolding and filaments that enable all cells to move around, change shape and divide. A cytoskeleton’s components act like tiny sticks and rubber bands that can expand, contract, materialize and disintegrate as needed. Networked together, they form dynamic structures, that are connected out to the points where a cell attaches to other surfaces. This lets the cell push, pull and climb around.
To study how the cytoskeleton works in GBM cells specifically, Kumar’s lab grows the tumor cells in different physical environments, including gels of varying stiffness and surfaces that are smooth or topographic, coated with different patterns of the proteins that the cells adhere to. In addition to tracking cell growth, movement, differentiation and division — standard biology — they perform structural analysis. They measure the tensile forces that the cell’s cytoskeleton exert and, to make the models even more accurate, they cut individual filaments within the cytoskeleton with precise lasers, which reveals the fiber’s individual contribution to the overall force that the cell exerts. These cuts also let them observe how the rest of the cytoskeletal elements shift around the newly broken link.
One of the findings from this work is that GBM tumor cells tend to contract in physically stiff environments, rather than expanding, dividing and floating off to spread elsewhere. This knowledge could lead to an effective treatment for brain cancer, but it wouldn’t be as simple as injecting a hardening agent into surgically removed tumor cavity, which isn’t safe. A more promising therapeutic approach is to use drugs that fool a cell into thinking that it’s in a stiff environment when it isn’t. Some such drugs, like hydroxyl fasudil and Y27632, have already entered the therapeutic pipeline against cancer without people fully understanding how they work. Kumar’s research pieces together the specific chain of chemical and physical reactions triggered by these drugs, and others, and the deeper understanding points to new ways of saving lives.
“We’re hoping to tie the two sides of it together,” Kumar sums up. “We want to contribute to therapies on the medical side and also figure out the underlying mechanisms, which requires lots of engineering approaches.”