Thermoelectrics: A matter of material
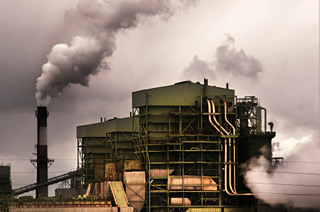
INEFFICIENT: In a coal-fired power plant, as much as 60 percent of the coal’s energy goes not to power homes as electricity but instead dissipates into the air, a problem called waste heat that several Berkeley researchers and entrepreneurs are working to solve.
Waste heat: It’s when heat produced in a combustive process goes unused, dissipating into the air or water. Automobiles, industrial facilities and power plants all produce waste heat, and a lot of it. In a coal-fired power plant, for example, as much as 60 percent of the coal’s energy doesn’t go to power homes as electricity but instead disappears into thin air, waste heat wasted.
For decades, that gross inefficiency has given the industry ulcers and lured others to try to capture waste heat before all that energy is lost. One solution has been an expensive boiler-turbine system, but for many companies and utilities, it doesn’t recover enough waste heat to make economic sense. Bismuth telluride, a semiconductor material, has shown promise by employing a thermoelectric principle called the Seebeck effect to convert heat into an electric current, but it’s also problematic: toxic, scarce and expensive, with limited efficiency.
While the search for clean, green energy has gotten gobs of attention recently, a holy grail awaits anyone who can improve the current fossil fuel system. One estimate places the worldwide waste heat recovery market at one trillion dollars, with the potential to offset as much as 500 million metric tons of carbon per year.
What’s the magic solution? Some Berkeley engineers believe the answer lies not in a sophisticated device, but in the basic elements of the periodic table—materials: specifically, finding a new material with spectacular thermoelectric properties that can efficiently and economically convert heat into electricity. (Thermoelectrics is the conversion of temperature differences, that is, differences in the amplitude of atom vibration in a solid, into an electric current.)
Over in Hearst Memorial Mining Building, materials science and engineering assistant professor Junqiao Wu may be onto something. Wu knew from earlier research that a good thermoelectric material needed to have a certain type of density of states, which is a mathematical description of distribution of energy levels within a semiconductor. “If the density of states is flat or gradual, the material won’t have very good thermoelectric properties,” Wu says. “You need it to be spiky or peaky.”
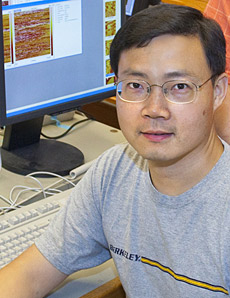
BETTER MATERIALS, BETTER ENGINEERING: Assistant professor Junqiao Wu of materials science and engineering is working on a new, highly efficient and low-cost thermoelectric material that may one day improve the performance of power plants, factories, cars and computers. (Photo by Junqiao Wu.)
Wu knew that a specialized type of semiconductor called highly mismatched alloys (HMAs) could be very peaky because of their hybridization, the result of forcing together two materials that don’t want to mix atomically, akin to mixing water and oil. He hypothesized that mixing two semiconductors, zinc selenide and zinc oxide, into an HMA, would produce a peaky density of states.
Beginning in late 2008, Wu collaborated with other researchers to run computations on “Franklin,” a massive Cray XT4 supercomputer at Lawrence Berkeley National Laboratory. After months of number crunching, Wu’s idea held up. Theoretically, at least, mixing the two materials enhanced their thermoelectric performance considerably, producing a new, highly efficient, potentially low-cost thermoelectric material. The physics journal Physical Review Letters published the team’s paper in January.
“We’re now working on experimentally synthesizing this material in the lab, doping it electrically [adding an impurity to make it conductive] and measuring the thermoelectric properties to prove what we predicted in our theory,” Wu explains. Forcing the two materials together in a uniform atomic pattern and keeping them together will be a challenging and potentially expensive process that could take three to five years. It might be another five years before Wu’s material is adapted into a thermoelectric device and sent out to recover waste heat.
“We see a very promising future for this material, but first we have to show that it works better than existing materials,” Wu says.
Wu isn’t the only Berkeley engineer in thermoelectrics. Materials science and engineering professors Ramamoorthy Ramesh, who holds a joint appointment with the physics department, and Peidong Yang, who holds a joint appointment with chemistry, are both pursuing various lines of query.
In fact, last year Yang cofounded a startup called Alphabet Energy with Berkeley Engineering alum Matt Scullin (M.S.’07, Ph.D.’09 MSE). Recently, Alphabet Energy received $1 million in seed money from Claremont Creek Ventures, a Berkeley venture capital firm, and CalCEF Clean Energy Angel Fund.
“We’re commercializing the first highly scalable, highly inexpensive thermoelectric platform that doesn’t require additional infrastructure,” Scullin says. “Factories, car makers, utilities, power plants, the military—they’re all interested in our technology.”
Another prominent Berkeley researcher in thermoelectrics is mechanical engineering and materials science and engineering professor Arun Majumdar, who now directs the U.S. Department of Energy’s Advanced Research Projects Agency–Energy (ARPA–E).
In February, ARPA–E awarded a $3 million grant to thermoelectric startup Phononic Inc., cofounded by Berkeley Engineering alum Patrick McCann (B.S.’81 Engineering Physics), who later earned his doctorate in electronic materials from MIT. McCann, an electrical and computer engineering professor at the University of Oklahoma, says work at Phononic Devices is based on semiconductor fabrication technology that he helped develop at Oklahoma.
“Thermoelectrics is a small world, and most good things seem to connect through UC Berkeley or MIT,” McCann says. Like Scullin and Yang, he is circumspect about revealing technical details but says, “We’re implementing known physical principles within a thermally insulating material to improve efficiency on a product that will be cost friendly to manufacturing.”
If these Berkeley engineers are successful, their thermoelectric materials may one day capture the energy that powers your home or boosts your car’s fuel efficiency. The breakthroughs may also cool the circuitry of electronics as large as server farms or as small as tiny portable devices. For Junqiao Wu and others working in the basic building blocks of Earth’s matter, all that magic is in the material.